In the dynamic fields of biotechnology and life sciences, the Fermentor Cell Culture Bioreator, Laboratory Fermenter Bioreactor, and Fermentation Bioreactor have emerged as indispensable tools, enabling scientists and researchers to unlock the potential of various biological processes.
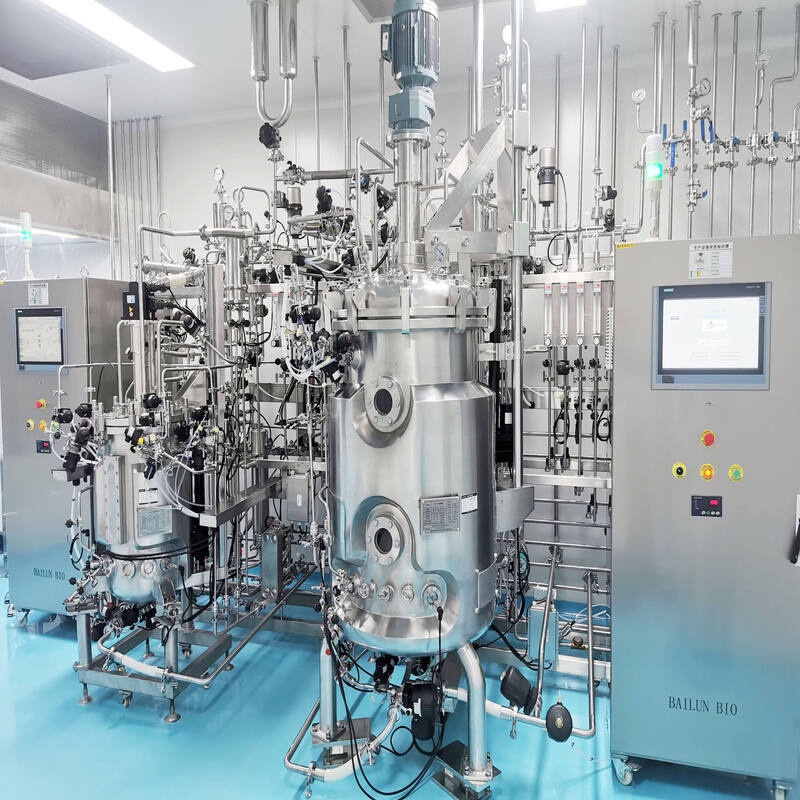
The Fermentor Cell Culture Bioreator plays a crucial role in the controlled growth and manipulation of cells. This sophisticated system provides an environment that closely mimics the natural conditions required for cells to thrive and perform their specific functions. By regulating parameters such as temperature, pH, dissolved oxygen levels, and nutrient supply, researchers can optimize cell growth and productivity.
For instance, in the production of therapeutic proteins using mammalian cell cultures, the precise control offered by the bioreactor is essential. It ensures that the cells produce the desired proteins in high quantities and with the correct post-translational modifications. This is critical for the efficacy and safety of the resulting biopharmaceuticals.
The Laboratory Fermenter Bioreactor serves as a vital tool in research and development settings. It allows scientists to conduct small-scale experiments and optimize fermentation conditions before scaling up to industrial production. This enables the exploration of different microbial strains, substrates, and process parameters to identify the most efficient and cost-effective combinations.
Consider the case of developing a novel microbial strain for the production of biofuels. Using the laboratory bioreactor, researchers can test various genetic modifications and cultivation strategies to enhance the yield and quality of the biofuel produced. The ability to make adjustments and monitor the effects in real-time provides valuable insights for process improvement.
The Fermentation Bioreactor, on a larger scale, is the backbone of industrial bioprocesses. It is responsible for the mass production of a wide range of products, from antibiotics and enzymes to food and beverage additives. The design and operation of these bioreactors are engineered to maximize productivity while maintaining strict quality control standards.
In the production of penicillin, for example, the fermentation bioreactor is carefully monitored and controlled to ensure the optimal growth of the penicillin-producing microorganisms. Precise control of parameters such as agitation speed, aeration rate, and substrate addition is necessary to achieve high yields and consistent product quality.
One of the key features of these bioreactor systems is their ability to handle different types of cultures and fermentation processes. They can accommodate microbial cultures such as bacteria, yeast, and fungi, as well as mammalian and plant cell cultures. This versatility makes them applicable in diverse areas of biotechnology, including healthcare, agriculture, and environmental science.
The monitoring and control systems associated with these bioreactors have also advanced significantly over time. Sensors and software now provide real-time data on various parameters, allowing for immediate adjustments and feedback control. This not only enhances the efficiency of the process but also reduces the risk of batch failures and product variations.
In addition to their role in production, 100l bioreactors also contribute to our understanding of fundamental biological processes. By studying how cells respond and adapt to different conditions within the controlled environment of the bioreactor, researchers can gain insights into cellular metabolism, gene expression, and regulatory mechanisms.
However, working with bioreactors is not without challenges. Maintaining aseptic conditions to prevent contamination is of utmost importance. Any introduction of foreign microorganisms or impurities can compromise the entire fermentation process and product quality. The sterilization of the bioreactor and its components, as well as the handling of raw materials and inoculums, require strict protocols and procedures.
The scalability of processes from the laboratory to industrial scale is another critical aspect. While results obtained in the laboratory provide a basis, translating these to large-scale production often involves addressing issues such as heat transfer, mass transfer, and shear stress effects that may not be significant at smaller scales.
Despite these challenges, the continued development and improvement of bioreactor technologies hold great promise. Emerging trends include the integration of single-use bioreactors for flexibility and reduced cleaning validation efforts, the application of advanced materials for improved biocompatibility and durability, and the use of synthetic biology approaches to engineer microorganisms for enhanced performance in the bioreactor environment.
In conclusion, the Fermentor Cell Culture Bioreator, Laboratory Fermenter Bioreactor, and Fermentation Bioreactor are at the forefront of modern biotechnology. Their ability to support controlled and efficient cell culture and fermentation processes has transformed the way we produce essential products, advance scientific research, and address global challenges in healthcare, industry, and the environment. As technology progresses, these bioreactor systems will undoubtedly continue to evolve, opening up new possibilities and shaping the future of bioprocess engineering.